NAVSTAR GPS SYSTEM EarthCache
-
Difficulty:
-
-
Terrain:
-
Size:
 (not chosen)
Please note Use of geocaching.com services is subject to the terms and conditions
in our disclaimer.
This earthcache is located in Como Lake Park and will explain the
Navstar GPS System and some of it's uses in the geosciences. I
STRONGLY SUGGEST YOU BRING A PRINTOUT OF THE CACHE PAGE WITH YOU!
When people talk about "a GPS," they usually mean a GPS receiver.
The Global Positioning System (GPS) is actually a constellation of
Earth-orbiting satellites with a minimum of 24 operational at all
times. The U.S. military developed and implemented this satellite
network as a military navigation system, but soon opened it up to
everybody else. Each of these 3,000- to 4,000-pound solar-powered
satellites circles the globe at about 12,000 miles, making two
complete rotations every day. The orbits are arranged so that at
any time, anywhere on Earth, there are at least four satellites
"visible" in the sky. A GPS receiver's job is to locate four or
more of these satellites, figure out the distance to each, and use
this information to deduce its own location. This operation is
based on a simple mathematical principle called trilateration.
Trilateration – Definition Trilateration is a method of
determining the relative positions of objects using the geometry of
triangles in a similar fashion as triangulation. Unlike
triangulation, which uses angle measurements (together with at
least one known distance) to calculate the subject's location,
trilateration uses the known locations of two or more reference
points, and the measured distance between the subject and each
reference point. To accurately and uniquely determine the relative
location of a point on a 2D plane using trilateration alone,
generally at least 3 reference points are needed Use In GPS
Receivers If you know you are 10 miles from satellite A in the sky,
you could be anywhere on the surface of a huge, imaginary sphere
with a 10-mile radius. If you also know you are 15 miles from
satellite B, you can overlap the first sphere with another, larger
sphere. The spheres intersect in a perfect circle. If you know the
distance to a third satellite, you get a third sphere, which
intersects with this circle at two points. The Earth itself can act
as a fourth sphere -- only one of the two possible points will
actually be on the surface of the planet, so you can eliminate the
one in space. Receivers generally look to four or more satellites,
however, to improve accuracy and provide precise altitude
information. In order to make this simple calculation, then, the
GPS receiver has to know two things: · The location of at
least three satellites above you · The distance between you
and each of those satellites The GPS receiver figures both of these
things out by analyzing high-frequency, low-power radio signals
from the GPS satellites. Better units have multiple receivers, so
they can pick up signals from several satellites simultaneously.
The GPS receiver calculates the distance to GPS satellites by
timing a signal's journey from satellite to receiver. As it turns
out, this is a fairly elaborate process. At a set time (let's say
midnight), each satellite begins transmitting a long, digital
pattern called a pseudo-random code. The receiver begins running
the same digital pattern also exactly at midnight. When the
satellite's signal reaches the receiver, its transmission of the
pattern will lag a bit behind the receiver's playing of the pattern
The length of the delay is equal to the signal's travel time. The
receiver multiplies this time by the speed of light to determine
how far the signal traveled. Assuming the signal traveled in a
straight line, this is the distance from receiver to satellite. In
order to make this measurement, the receiver and satellite both
need clocks that can be synchronized down to the nanosecond. To
make a satellite positioning system using only synchronized clocks,
you would need to have atomic clocks not only on all the
satellites, but also in the receiver itself. But atomic clocks
would be just a bit too expensive for everyday consumer use. The
Global Positioning System has a clever, effective solution to this
problem. Every satellite contains an expensive atomic clock, but
the receiver itself uses an ordinary quartz clock, which it
constantly resets. In a nutshell, the receiver looks at incoming
signals from four or more satellites and gauges it’s own
inaccuracy. In other words, there is only one value for the
"current time" that the receiver can use. The correct time value
will cause all of the signals that the receiver is receiving to
align at a single point in space. That time value is the time value
held by the atomic clocks in all of the satellites. So the receiver
sets its clock to that time value, and it then has the same time
value that all the atomic clocks in all of the satellites have. The
GPS receiver gets atomic clock accuracy "for free." When you
measure the distance to four located satellites, you can draw four
spheres that all intersect at one point. Three spheres will
intersect even if your numbers are way off, but four spheres will
not intersect at one point if you've measured incorrectly. Since
the receiver makes all its distance measurements using its own
built-in clock, the distances will all be proportionally incorrect.
The receiver can easily calculate the necessary adjustment that
will cause the four spheres to intersect at one point. Based on
this, it resets its clock to be in sync with the satellite's atomic
clock. The receiver does this constantly whenever it's on, which
means it is nearly as accurate as the expensive atomic clocks in
the satellites. In order for the distance information to be of any
use, the receiver also has to know where the satellites actually
are. This isn't particularly difficult because the satellites
travel in very high and predictable orbits. The GPS receiver simply
stores an almanac that tells it where every satellite should be at
any given time. Things like the pull of the moon and the sun do
change the satellites' orbits very slightly, but the Department of
Defense constantly monitors their exact positions and transmits any
adjustments to all GPS receivers as part of the satellites'
signals. To log this cache, you must complete the following steps.
1. Starting at the posted coordinates, you will see a road that
leads to the Bowen Rd. Entrance of the park. Your task is to follow
that road and collect the following information and email it to me;
a. The coordinates AND elevation of the highest and lowest points
along the road. b. The coordinates AND elevation of the start and
end point of the road (use the stop signs as the start and end
points. DO NOT POST THIS INFORMATION IN YOUR LOG! 2. Post a photo
of yourself, or your group, with your GPS unit/units at the park
entrance sign near Bowen Rd. Although there are many uses for GPS
information in the geosciences, I will explain one, and have you
perform another. Hayward Fault GPS Campaign The Hayward fault is
unique because it not only produces large earthquakes like the
estimated M7 1868 earthquake, but it also slowly slips along in a
process called aseismic creep. In recent years, the Berkeley
Seismological Laboratory has begun utilizing measurements with the
Global Positioning System (GPS) to monitor creep along the Hayward
fault. This work will provide an unprecedented spatial resolution
of GPS measurements about a creeping fault and should allow us to
determine where the fault is creeping, how fast it is creeping, and
most importantly, how deep the creep extends. Since aseismic creep
relieves stress along the fault, identifying locked patches at
depth can give us clues to determine where large earthquakes might
eventually nucleate and how big they could be. The researchers use
research-grade GPS equipment to determine the location of a point
on the ground to a precision of less than 5 mm. In other words,
they can determine locations on earth to within an area half the
size of a dime. The next year, they repeat the campaign and return
to the exact same location to determine the position again. Because
the Hayward fault is slowly creeping along, the spot will have
moved; with high precision positioning, they hope to determine
exactly where and how fast.. As part of the Berkeley Seismological
Laboratory's ongoing effort to monitor active deformation along the
Hayward fault, the researchers determine positions of over fifty
benchmarks within ten kilometers of the fault. The first surveys of
most benchmarks were made in 1998, and their group has been
returning each summer to take new measurements. These observations
show that the Hayward fault creeps at a rate of about 4.2 mm per
year.
I have earned GSA's highest level: |
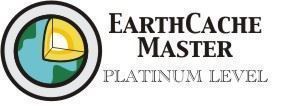 |
Additional Hints
(No hints available.)